INTRODUCTION
Construction of masonry wall systems is possible without the use of mortar. The use of standard CMU units laid dry and subsequently surface bonded with fiber reinforced surfaced bonding cement has been well documented in the past. (ref. 16) With the use of specially fabricated concrete masonry units known as “dry-stack units,” construction of these mortarless systems is simple, easy and cost effective. This TEK describes the construction and engineering design of such mortarless wall systems.
The provisions of this TEK apply to both specialty units manufactured specifically for dry-stack construction and conventional concrete masonry units with the following system types:
- Grouted, partially grouted or surface bonded
- Unreinforced, reinforced, or prestressed
Note that dry-stacked prestressed systems are available that do not contain grout or surface bonding. The provisions of this TEK do not apply to such systems due to a difference in design section properties (ref 8).
Specially designed units for dry-stack construction are available in many different configurations as shown in Figure 1. The latest and most sophisticated designs incorporate face shell alignment features that make units easier and faster to stack plumb and level. Other units are fabricated with a combination of keys, tabs or slots along both horizontal and vertical faces as shown in Figure 1 so that they may interlock easily when placed. Physical tolerances of dry-stack concrete units are limited to ±1/16 in. (1.58 mm.) which precludes the need for mortaring, grinding of face shell surfaces or shimming to even out courses during construction. Interlocking units placed in running bond resist flexural and shear stresses resulting from out-of-plane loads as a result of the keying action: (a) at the top of a web with the recess in the web of the unit above, (b) at two levels of bearing surface along each face shell at the bed joint, and (c) between adjacent blocks along the head joint. The first of these two interlocking mechanisms also ensures vertical alignment of blocks.
The interlocking features of dry-stack units improve alignment and leveling, reduce the need for skilled labor and reduce construction time. Floor and roof systems can be supported by mortarless walls with a bond beam at the top of the wall which expedites the construction process.
Wall strength and stability are greatly enhanced with grouting which provides the necessary integrity to resist forces applied parallel, and transverse to, the wall plane. Vertical alignment of webs ensures a continuous grout column even when the adjacent cell is left ungrouted. Grouting is necessary to develop flexural tensile stress normal to the bed joints, which is resisted through unit-mortar bond for traditional masonry construction. Strength of grouted dry-stack walls may also be enhanced by traditional reinforcement, prestressing, post-tensioning or with external fiber-reinforced surface coatings (surface bonding) as described in the next section.
Typical applications for mortarless concrete masonry include basement walls, foundation walls, retaining walls, exterior above-grade walls, internal bearing walls and partitions. Dry-stack masonry construction can prove to be a cost-effective solution for residential and low-rise commercial applications because of it’s speed and ease of construction, strength and stability even in zones of moderate and high seismicity. More information on design and construction of dry-stack masonry can be found in Reference 5.
CONSTRUCTION
Dry-stack concrete masonry units can be used to construct walls that are grouted or partially grouted; unreinforced, reinforced or prestressed; or surface bonded. With each construction type, walls are built by first stacking concrete masonry units.
For unreinforced construction as shown in Figure 2a, grouting provides flexural and shear strength to a wall system. Flexural tensile stresses due to out-of-plane bending are resisted by the grout cores. Grout cores also interlace units placed in running bond and thus provide resistance to in-plane shear forces beyond that provided by friction developed along horizontal joints. Grout cores can also be reinforced to increase flexural strength.
Reinforcement can be placed vertically, in which case only those cells containing reinforcement may be grouted as shown in Figure 2b, as well as horizontally, in which case the masonry must be fully grouted. Another version is to place vertical prestressing tendons in place of reinforcement. Vertical axial compressive stress, applied via the tendons, increases flexural and shear capacity. Tendons may be bonded to grout, or unbonded, based upon the design. Placement of grout may be optional. Horizontally reinforced bond beam lintels can be created using a grout stop beneath the unit to contain grout.
As an alternative to reinforcing or prestressing, wall surfaces may be parged (coated) with a fiber-reinforced surface bonding cement/stucco per ASTM C887(ref. 14) as illustrated in Figure 2c. This surface treatment, applied to both faces of a wall, bonds concrete units together without the need for grout or internal reinforcement. The parging material bridges the units and fills the joints between units to provide additional bonding of the coating to the units through keying action. The compressive strength of the parging material should be equal to or greater than that of the masonry units.
Laying of Units
The first course of dry-stack block should be placed on a smooth, level bearing surface of proper size and strength to ensure a plumb and stable wall. Minor roughness and variations in level can be corrected by setting the first course in mortar. Blocks should be laid in running bond such that cells will be aligned vertically.
Grout and Reinforcement
Grout and grouting procedures should be the same as used in conventional masonry construction (ref. 1, 10) except that the grout must have a compressive strength of at least 2600 psi (190 MPa) at 28 days when tested in accordance with ASTM C 1019 (ref.12). Placement of grout can be accomplished in one lift for single-story height walls less than 8 ft (2.43 m). Grout lifts must be consolidated with an internal vibrator with a head size less than 1 in. (25 mm).
Vertical Reinforcing
As for conventional reinforced masonry construction, good construction practice should include placement of reinforcing bars around door and window openings, at the ends, top and bottom of a wall, and between intersecting walls. Well detailed reinforcement such as this can help enhance nonlinear deformation capacity, or ductility, of masonry walls in building systems subjected to earthquake loadings – even for walls designed as unreinforced elements. Additional information on conventional grouting and reinforced masonry wall can be found in TEK 09-04A and TEK 03-03B (refs. 9 & 6).
Pre-stressed Walls
Mortarless walls can also be prestressed by placing vertical tendons through the cores. Tendons can be anchored within the concrete foundation at the base of a wall or in a bottom bond beam and are tensioned from the top of a wall.
Surface Bonded Walls
For walls strengthened with a surface bonding, a thin layer of portland cement surface bonding material should be troweled or sprayed on to a wall surface. The thickness of the surface coating should be at least ⅛ in. (3.2 mm.) or as required by the material supplier.
ENGINEERING PROPERTIES
Walls constructed with mortarless masonry can be engineered using conventional engineering principles. Existing building code recommendations such as that produced by the building code (ref. 1) can serve as reference documents, but at the time of this printing it does not address mortarless masonry directly. It is thus considered an alternate engineered construction type. The International Building Code (ref. 7) does list allowable stresses based on gross-cross-sectional area for dry-stacked, surface-bonded concrete masonry walls. These values are the same as presented in TEK 03-05A (ref. 16). Suggested limits on wall or building height are given in Table 1.
Test data (refs. 2, 3 and 4) have shown that the strength of drystack walls exceeds the strength requirements of conventional masonry, and thus the recommended allowable stress design practices of the code can be used in most cases. When designing unreinforced, grouted masonry wall sections, it is important to deduct the thickness of the tension side face shell when determining the section properties for flexural resistance.
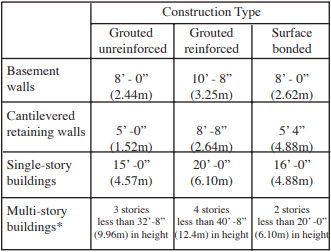
* Laterally supported at each floor
Unit and Masonry Compressive Strength
Units used for mortarless masonry construction are made of the same concrete mixes as used for conventional masonry units. Thus, compressive strength of typical units could vary between 2000 psi (13.79MPa) and 4000 psi. (27.58 MPa) Standard Methods of Sampling and Testing Concrete Masonry Units (ref. 11) can be referred to for determining strength of dry-stack units.
Masonry compressive strength f’m can conservatively be based on the unit-strength method of the building code (ref . 15), or be determined by testing prisms in accordance with ASTM C1314 (ref. 4). Test prisms can be either grouted or ungrouted depending on the type of wall construction specified.
Solid Grouted, Unreinforced Construction
Out-of-Plane & In-Plane Allowable Flexural Strength
Because no mortar is used to resist flexural tension as for conventional masonry construction, flexural strength of mortarless masonry is developed through the grout, reinforcement or surface coating. For out-of-plane bending of solid grouted walls allowable flexural strength can be estimated based on flexural tensile strength of the grout per Equation 1.
Consideration should be given to the reduction in wall thickness at the bed joints when estimating geometrical properties of the net effective section.
Correspondingly, flexural strength based on masonry compressive stress should be checked, particularly for walls resisting significant gravity loads, using the unity equation as given below.
Buckling should also be checked. (Ref. 8)
In-Plane Shear Strength
Shear strength for out-of-plane bending is usually not a concern since flexural strength governs design for this case. For resistance to horizontal forces applied parallel to the plane of a wall, Equation 3 may be used to estimate allowable shear strength.
Fv is the allowable shear strength by the lesser of the three values given in Equation 4.
Grouted, Reinforced Construction
Mortarless masonry that is grouted and reinforced behaves much the same as for conventional reinforced and mortared construction. Because masonry tensile strength is neglected for mortared, reinforced construction, flexural mechanisms are essentially the same with or without the bed joints being mortared provided that the units subjected to compressive stress are in good contact. Thus, allowable stress design values can be determined using the same assumptions and requirements of the MSJC code. (ref.1)
Out-of-Plane & In-Plane Allowable Flexural Strength
Axial and flexural tensile stresses are assumed to be resisted entirely by the reinforcement. Strains in reinforcement and masonry compressive strains are assumed to vary linearly with their distance from the neutral axis. Stresses in reinforcement and masonry compressive stresses are assumed to vary linearly with strains. For purposes of estimating allowable flexural strengths, full bonding of reinforcement to grout are assumed such that strains in reinforcement are identical to those in the adjacent grout.
For out-of-plane loading where a single layer of vertical reinforcement is placed, allowable flexural strength can be estimated using the equations for conventional reinforcement with the lower value given by Equations 5 or 6.
In-Plane Shear Strength
Though the MSJC code recognizes reinforced masonry shear walls with no shear, or horizontal reinforcement, it is recommended that mortarless walls be rein- forced with both vertical and horizontal bars. In such case, allowable shear strength can be determined based on shear reinforcement provisions (ref. 1) with Equations 7, 8 and 9.
Where Fv is the masonry allowable shear stress per Equations 8 or 9.
Solid Grouted, Prestressed Construction
Mortarless masonry walls that are grouted and pre- stressed can be designed as unreinforced walls with the prestressing force acting to increase the vertical compres- sive stress. Grout can be used to increase the effective area of the wall. Flexural strength will be increased because of the increase in the fa term in Equation 1. Shear strength will be increased by the Nv term in Equation 4.
Because the prestressing force is a sustained force, creep effects must be considered in the masonry. Research on the long-term behavior of dry-stacked masonry by Marzahn and Konig (ref. 8) has shown that creep effects may be accentuated for mortarless masonry as a result of stress concentrations at the contact points of adjacent courses. Due to the roughness of the unit surfaces, high stress concentrations can result which can lead to higher non-proportional creep deformations. Thus, the creep coefficient was found to be dependent on the degree of roughness along bed-joint surfaces and the level of applied stress. As a result, larger losses in prestressing force is probable for dry-stack masonry.
Surface-Bonded Construction
Dry-stack walls with surface bonding develop their strength through the tensile strength of small fiberglass fibers in the 1/8” (3.8mm) thick troweled or surface bonded cement-plaster coating ASTM C-887(Ref. 14). Because no grouting is necessary, flexural tension and shear strength are developed through tensile resistance of fiberglass fibers applied to both surfaces of a wall. Test data has shown that surface bonding can result in a net flexural tension strength on the order of 300 psi.(2.07 MPa) Flexural capacity, based on this value, exceeds that for conventional, unreinforced mortared masonry construction, therefore it is considered conservative to apply the desired values of the code (ref. 1) for allowable flexural capacity for portland cement / lime type M for the full thickness of the face shell.
Out-of-Plane and In-Plane Flexural Strength
Surface-bonded walls can be considered as unreinforced and ungrouted walls with a net allowable flexural tensile strength based on the strength of the fiber-reinforcement. Flexural strength is developed by the face shells bonded by the mesh. Allowable flexural strength can be determined using Equation 1 with an Ft value determined on the basis of tests provided by the surface bonding cement supplier. Axial and flexural compressive stresses must also be checked per Equation 2 considering again only the face shells to resist stress.
Surface Bonded In-Plane Shear Strength
In-plane shear strength of surface-bonded walls is attributable to friction developed along the bed joints resulting from vertical compressive stress in addition to the diagonal tension strength of the fiber coating. If the enhancement in shear strength given by the fiber reinforced surface parging is equal to or greater than that provided by the mortar-unit bond in conventional masonry construction, then allowable shear strength values per the MSJC code (ref. 1) may be used. In such case, section properties used in Equation 3 should be based on the cross-section of the face shells.
REFERENCES
- Building Code Requirements for Masonry Structures), ACI 530-02/ ASCE 5-02/TMS 402-02. Reported by the Masonry Standards Joint Committee (MSJC), 2002.
- Drysdale, R.G., Properties of Dry-Stack Block, Windsor, Ontario, July 1999.
- Drysdale, R.G., Properties of Surface-Bonded Dry-Stack Block Construction, Windsor, Ontario, January 2000.
- Drysdale, R.G., Racking Tests of Dry-Stack Block, Windsor, Ontario, October 2000.
- Drysdale, R.G., Design and Construction Guide for Azar Dry-Stack Block Construction, JNE Consulting, Ltd., February 2001.
- Grout for Concrete Masonry, TEK 09-04A, Concrete Masonry & Hardscapes Association, 2002.
- 2000 International Building Code, Falls Church, VA. International Code Council, 2000.
- Marzahn, G. and G. Konig, Experimental Investigation of Long-Term Behavior of Dry-Stacked Masonry, Journal of The Masonry Society, December 2002, pp. 9-21.
- Hybrid Concrete Masonry Construction Details, TEK 0303B. Concrete Masonry & Hardscapes Association, 2009.
- Specification for Masonry Structures, ACI 530.1-02/ASCE 6-02/ TMS 602-02. Reported by the Masonry Standards Joint Committee (MSJC), 2002.
- Standard Methods of Sampling and Testing Concrete Masonry Units, ASTM C140-02a, ASTM International, Inc. , Philadelphia, 2002.
- Standard Method of Sampling and Testing Grout, ASTM C1019-02, ASTM International, Inc., Philadelphia, 2002.
- Standard Specification for Grout for Masonry, ASTM C 476-02. ASTM International, Inc., 2002
- Standard Specification for Packaged, Dry, Combined Materials for Surface Bonding Mortar, ASTM C 887-79a (2001). ASTM International, Inc., 2001.
- Standard Test Method for Compressive Strength of Masonry Assem blages, ASTM C1314-02a, ASTM International, Inc., Philadelphia, 2002.
- Surface Bonded Concrete Masonry Construction, TEK 03-05A. Concrete Masonry & Hardscapes Association, 1998.
NOTATION
An net cross-sectional area of masonry, in² (mm²)
As effective cross-sectional area of reinforcement, in2 (mm2)
b width of section, in. (mm)
d distance from extreme compression fiber centroid of tension reinforcement, in. (mm)
Fa allowable compressive stress due to axial load only, psi (MPa)
Fb allowable compressive stress due to ß exure only, psi (MPa)
Fs allowable tensile or compressive stress in reinforcement, psi (MPa)
Ft flexural tensile strength of the grout, psi(MPa)
Fv allowable shear stress in masonry psi (MPa)
fa calculated vertical compressive stress due to axial load, psi (MPa)
fb calculated compressive stress in masonry due to ß exure only, psi (MPa)
f’ specified compressive strength of masonry, psi (MPa)
I moment of inertia in.4 (mm4)
j ratio of distance between centroid of flexural compressive forces and centroid of tensile forces to depth, d
k ratio of the distance between compression face of the wall and neu tral axis to the effective depth d
M maximum moment at the section under consideration, in.-lb (N-mm)
Nv compressive force acting normal to the shear surface, lb (N)
Q first moment about the neutral axis of a section of that portion of the cross section lying between the neutral axis and extreme fiber in.³ (mm³)
Sg section modulus of uncracked net section in.³ (mm³)
V shear force, lb (N)