INTRODUCTION
Passive solar design involves utilizing a building’s basic elements walls, windows and floors—to produce a comfortable environment with less reliance on mechanical heating and cooling. Passive solar systems can provide space heating, natural ventilation, cooling load avoidance, daylighting and water heating. The U. S. Department of Energy estimates that 30 to 50% energy cost reductions are economically realistic in new office design with an optimum mix of energy conservation and passive solar design strategies (ref. 1). In addition, most passive solar design strategies integrate well with active solar applications such as photovoltaics.
Concrete masonry plays a vital role in effective passive solar design, by providing thermal mass to absorb and slowly release solar heat. Without sufficient thermal mass, passive solar buildings can overheat and be uncomfortable.
It is most economical to evaluate passive solar strategies early in the design process. The rules of thumb included in this TEK are intended as a starting point for determining preliminary size and location for concrete masonry and glazing. As the design progresses, a more detailed analysis should be performed, preferably using software designed to accommodate passive solar interactions. Some appropriate software programs are briefly discussed near the end of this TEK.
PASSIVE SOLAR IN BUILDING CODES AND LEED
Renewable energy sources, such as passive solar, are typically not explicitly included in energy code criteria. Often, a passive solar building will comply with energy code requirements when the passive solar elements are neglected. Where this is not the case, for example where the prescriptive limit on glazing area is exceeded, most building codes allow compliance using an analysis based on whole building performance. For residential buildings three stories or less in height, Chapter 4 of the International Energy Conservation Code (IECC, ref. 2) describes the criteria for an annual energy analysis to demonstrate compliance.
For commercial and high-rise residential buildings, IECC compliance falls under section 806, Total Building Performance, or via Chapter 7 which references Energy Standard for Buildings Except Low-Rise Residential Buildings, ASHRAE/IESNA 90.1-2001 (ref. 3). Section 11 of Standard 90.1, Energy Cost Budget Method, is used for passive solar compliance. Note that a 2004 edition of ASHRAE 90.1 is also available, but the energy cost budget provisions are essentially the same as those in the 2001 edition. As for IECC Chapter 4, these sections define criteria for the annual whole-building analysis required to demonstrate compliance.
In the LEED program (ref. 4), passive solar systems are included under Energy and Atmosphere Credit 1, Optimize Energy Performance. This credit again references the energy cost budget method defined in Section 11 of ASHRAE/IESNA Standard 90.1 for demonstrating the building energy performance. Note that E&A Credit 2, Renewable Energy, applies only to renewable energy systems that generate power, such as photovoltaics, biomass and wind turbines.
ELEMENTS OF A PASSIVE SOLAR DESIGN
The components of a passive solar design are familiar parts of any building. In a passive solar building, however, these elements are carefully chosen, sized and located to work together to provide comfort. The function of each of these components is briefly described here. Some general guidance regarding sizing and location is included in the section Passive Solar Design Rules of Thumb.
Thermal Mass
Thermal mass in passive solar design provides three functions: it quickly absorbs solar heat to help avoid overheating when the sun shines; it stores the solar heat; and it slowly releases the heat to provide warmth after the sun sets. Concrete masonry walls and concrete paver floors are very efficient thermal storage mediums, and are commonly used in passive solar buildings to provide these functions. Masonry location and thickness are important to passive solar design, as are the conduction, specific heat and density. As these three properties increase, the heat storage effectiveness generally increases accordingly.
However, very high conductances should be avoided since this can shorten the time lag for heat delivery.
One important performance measure of passive solar buildings is the ability to maintain comfortable indoor temperatures. The amplitude of the indoor temperature swing is determined by the amount of effective thermal mass in the building. As the amount of thermal mass increases, the daily indoor temperature swing typically decreases.
Glazing
Glazing allows solar heat and light into the building. Choice of particular glazing products, sizes and locations will vary with the desired heat gain, cooling load avoidance and daylighting needs. These may vary within the building according to how the interior spaces are used. For example, because of glare, areas such as lobbies and atria may be more appropriate on a south-facing wall with a large amount of direct sunlight, than, for example, an office space.
Shading
Appropriate shading helps prevent solar heat gain during the summer. Shading may include permanent overhangs or porch roofs, moveable awnings, shutters, vegetation to shade east and west-facing windows, and/or limiting east/west glass.
Ventilation
Venting can rid the building of heat when the thermal mass is saturated. It can also provide outdoor air to cool the building when the outside air is cooler than the building’s thermostat setting, such as by precooling the building at night. Ventilation can be accomplished using natural ventilation or using an exhaust fan tied to a thermostatic control.
Types of Passive Solar Designs
Passive solar designs can generally be classified as one of three types, depending on where the solar heat is collected relative to where it is used: direct gain, indirect gain or isolated gain. The basic components are illustrated in Figure 1.
Direct Gain Systems
In a direct gain space, solar energy penetrates directly into the space where it is stored and used. Direct gain systems are the simplest to install since only windows and mass are required. Figure 1a shows the proper use of thermal mass in the walls and on the floor. Heat is collected and distributed by transmission through the windows, absorption at the mass surface, and convection and radiation within the room. Using sufficient thermal mass improves performance and comfort.
Indirect Gain
With indirect gain, a thermal storage material is used between the glazing and the space to be heated to collect, store and distribute solar radiation. An example is the trombe wall (see Figure 1b). A trombe wall uses a south-facing masonry wall faced with glazing placed 3/4 to 2 in. (19-51 mm) from the masonry. Heat from sunlight passing through the glass is absorbed by the masonry and slowly transferred through the wall to the interior space. Shading and/or ventilation are used to prevent unwanted heat gains during warmer periods. Vents at the top and bottom of a trombe wall are sometimes included to set up a convective current for passive cooling.
Isolated Gain
Isolated gain systems, such as sunspaces, collect solar energy in an area that can be closed off from the rest of the building. In addition to thermal mass floors, sunspaces typically use concrete masonry walls for thermal storage and as a heat transfer “valve” between the sunspace and the living or working space. Sunspace heat can be moved through vents with backdraft dampers to prevent improper flow. A fan, doors and/or windows can also be used to circulate warm air to the living space.
Because of the potential for overheating, care must be used when designing with sloped (i.e., overhead) glazings. Vertical glazings and pop-up skylights can be used with only a small decrease in performance. Vertical glazing is less expensive than sloped glazing, and overheating is more easily prevented.
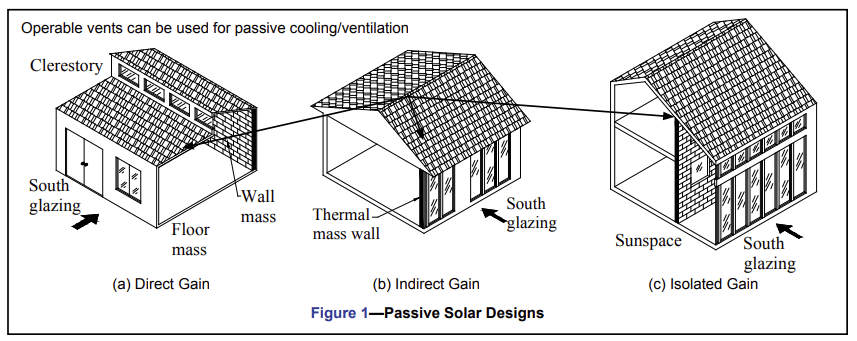
PASSIVE SOLAR DESIGN RULES OF THUMB
Rules of thumb are useful early in the design process, as a first step in laying out the building and sizing the systems and materials. The rules of thumb listed below are most appropriate for buildings with skin-dominated heating loads, such as residential and small commercial buildings. Note that many of these design considerations involve compromises. For example, allowing maximum solar heat gain while minimizing summer heat gain. The designer should consider the specifics of the site and climate when evaluating appropriate passive solar design strategies. Software is particularly effective for evaluating these interactions for a particular building. General suggestions for successful passive solar performance include:
- Building orientation. Ideally, the building south wall should face within 15 degrees of true south. With this orientation, the building receives maximum winter and minimum summer heat gains. Between 15 and 30 degrees east or west of true south, performance tends to be reduced about 15 percent from the optimum.
- Buffer the north side of the building. Place rooms with low heating, lighting and use requirements, such as utility rooms, storage rooms, and garages on the north side of the building to buffer the other spaces. This can reduce the normally higher heat loss through north walls while not interfering with solar access.
- Match the solar heating system to the room use. Consider occupancy patterns when choosing a system: what are the heating, daylighting and privacy requirements of the room? For example, a bedroom requires privacy and needs heat after sunset, so a thermal storage wall might be the logical choice. A living room, on the other hand, needs daytime and early evening heat and has higher lighting requirements; therefore, a direct gain system or sunspace may be more appropriate.
- Include adequate thermal mass. For buildings with southfacing glass area more than 7% of the floor area, additional thermal mass must be included to absorb heat and maintain comfort. Thermal mass, such as concrete masonry walls and concrete paver floors, should be relatively thin (2 to 4-in. (51 to 102-mm) thick) to allow heat absorption and release within a 24-hour cycle; and should be spread over a large area to help prevent localized hot or cold spots.
Eight-in. (203-mm) fully grouted concrete masonry should be used if the wall is used as a north-south division wall separating two direct gain rooms. Such a wall can store heat on both sides, optimizing the mass storage. Do not fill the cores of these walls with sand, soil or insulation.
For trombe walls, the concrete masonry wall is typically 8 to 16 in. (203 to 406 mm) thick, depending on the desired time lag for heat distribution indoors.
Minimum recommended ratios of thermal mass area to additional glass area (i.e., south-facing glass area > 7%) are (ref. 5):
- 1: 5.5 for floor in direct sunlight,
- 1:4 for floor not in direct sunlight, and
- 1:8.3 for wall and ceilings.
5. Distribute the thermal mass throughout the room. In direct gain systems, the primary collection mass is placed in direct sunlight. In addition to this mass, comfort is improved if mass is distributed evenly around the room because localized hot or cold spots are less likely to develop. Performance is relatively the same whether the mass is located on the east, west or north walls, or in the floor. The mass should be distributed over an interior surface area approximately equal to six times the solar glass area.
6. Avoid “insulating” thermal mass. Rugs or carpets in the solar collection space will significantly impact thermal mass performance. In general, an exposed strip of massive floor about 8 ft (2.4 m) wide provides a good floor collection area.
7. Select an appropriate thermal mass color. Masonry walls can be any color in direct gain systems, although the mass should be somewhat darker (0.5 < a < 0.8) than the low-mass materials. (Absorptivity, a, ranges from 0 to 1, indicating the percentage of incident solar energy that is absorbed. a = 1 indicates 100% absorption) The absorptivity of natural or colored concrete masonry falls in this range without paints or special treatments. Mass walls that are too dark (0.8 < a < 1.0), can result in high surface temperatures where surfaces are exposed directly to the sun, and less absorption elsewhere on the wall. Masonry floors, on the other hand, should be dark (0.7 < a < 1.0) to increase the absorption of the concrete pavers or masonry units and to counter the tendency of the heat to be released too rapidly to the atmosphere. A matt floor finish will maximize absorption and reduce glare. Thermal storage walls should be dark (a > 0.8) or coated with a selective surface material, such as a metallic film specifically designed to maximize absorption and minimize heat loss due to radiation back towards the glass. Materials without significant thermal mass, such as frame walls, should be lighter in color.
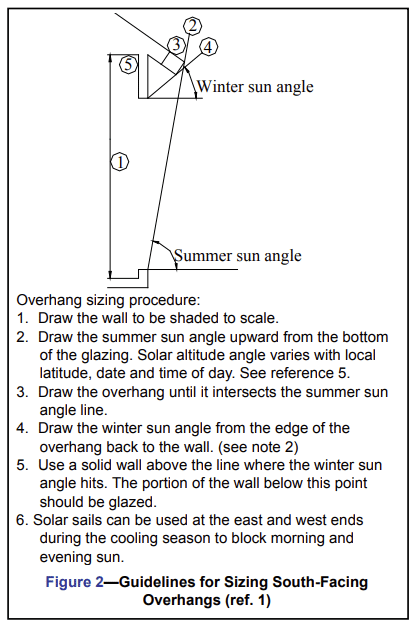
- Choose appropriate glass. Windows are typically rated by their solar heat gain coefficient (SHGC) and conductance (U-factor). Regardless of climate, the U-factor should be as low as possible (0.35 or less), to minimize conductive heat loss through the windows. For south-facing glass in most climates, choose windows with a SHGC as high as possible (0.6 or higher) to allow maximum heat gain during the winter, and rely on overhangs or other shading to limit summer sun. In cooling climates, such as south Florida, choose windows with a SHGC as low as possible.
- Use appropriate window shading. Windows facing within about 30o of true south can be shaded with properly sized overhangs. Figure 2 shows guidelines for sizing the overhangs to allow sunlight entry from about mid September through mid-March (software is also available for sizing overhangs). Off-south wall orientations reduce overhang effectiveness.
East- and west-facing glass can be a significant source of heat gain and glare year-round, because of low morning and evening sun angles. Of the two, west-facing glass is more of a concern, because afternoons are typically hotter than mornings. There are several strategies to address these issues: limit the area of east- and west-facing glass; use wide overhangs (such as porch roofs; smaller overhangs will not be effective); use evergreens for shading; or use glass that blocks solar heat (although this may require different glass types for different walls of the building). - Landscaping. Consider solar access and prevailing wind patterns when choosing trees and shrubs. Issues to consider include: shading of south-, east- and west-facing glass; channeling summer breezes; summer shading of the roof and paved areas; and blocking prevailing winter winds. Because leafless deciduous trees can block as much as 30% of winter solar energy, trees should not be placed where they block the south-facing windows in locations where significant winter solar heating is expected. However, in climates where summer heat is a significant problem, trees on the south-facing side may be appropriate.
SOFTWARE TOOLS
Software to evaluate passive solar buildings should include an annual whole-building analysis, and be able to correctly model solar gains and thermal mass. Programs such as DOE2 and BLAST are very comprehensive and well-documented. However, these programs require a high level of user expertise and can be cumbersome to use.
Energy-10 is a conceptual design tool focused on making whole building trade-offs during early design phases for residential and small commercial buildings (less than 10,000 ft2 (930 m2 ) floor area). The program performs an hourly annual whole-building energy analysis, including dynamic thermal and daylighting calculations. Outputs include a summary table, detailed tabular results and 20 graphical outputs.
EnergyPlus builds on the capabilities of BLAST and DOE2, with some additional simulation capabilities such as time steps of less than an hour. Input and output are via ASCII text files, although the program was developed to facilitate thirdparty user-friendly interfaces (see http://www.eere.energy.gov/ buildings/energyplus/ for available interfaces).
These programs are described on the Department of Energy website, http://www.eere.energy.gov/buildings/tools_directory. The website contains a list of over 300 building analysis programs, searchable by name, subject or platform. The site also includes information on program capabilities, input, output, user expertise required, how to obtain the software, as well as strengths and weaknesses.
REFERENCES
- Passive Solar Design. U. S. Department of Energy, www.eere.energy.gov/buildings/info/design/integratedbuilding/passive.html.
- 2003 International Energy Conservation Code. International Code Council, 2003.
- Energy Standard for Buildings Except Low-Rise Residential Buildings, ASHRAE/IESNA 90.1-2001/2004. American Society of Heating, Refrigerating and AirConditioning Engineers, Inc., 2001/2004.
- LEED for New Construction and Major Renovations, LEED-NC version 2.2. U. S. Green Building Council, 2005.
- Green Building Guidelines: Meeting the Demand for LowEnergy, Resource-Efficient Homes. Sustainable Buildings Industry Council, 2004.
- ASHRAE Handbook, Fundamentals. American Society of Heating, Refrigerating and Air-Conditioning Engineers, Inc., 2005.